2018 IBC Analyzing Key Structural Design Updates for Enhanced Building Safety
2018 IBC Analyzing Key Structural Design Updates for Enhanced Building Safety - Deflection Limits for Metal Roofing and Siding
The 2018 International Building Code (IBC) establishes specific deflection limits for metal roofing and siding systems, a key update aimed at boosting structural soundness and visual appeal. The code mandates that the total deflection under load for formed metal sheets must remain within a set span limit (l). This same span limit also governs the deflection of secondary framing members—those supporting either metal roofs under live loads or metal siding under design wind loads. These deflection limits aren't simply about meeting code requirements; they are fundamental to maintaining the integrity and appearance of metal roofing and siding installations.
Chapter 15 of the IBC further underscores the importance of well-designed roof assemblies, emphasizing the crucial role of weather-resistant and fire-resistant barriers. The IBC's focus on deflection, along with these additional requirements, demonstrates a broader push for enhanced safety in building design and construction, specifically within the context of metal roof and siding applications. These updates are a recognition of how advancements in materials and design have changed building practices, necessitating a more stringent approach to ensure structural performance and reliability. While historically, deflection limitations were tied to structural safety, the 2018 IBC acknowledges their visual implications, as well, promoting a holistic perspective on metal building designs.
The 2018 International Building Code (IBC) establishes deflection limits for metal roofing and siding, often expressed as a fraction of the span length (L), like L/180 or L/240. This signifies the maximum allowable flex before the structure's functionality or appearance is compromised. Temperature changes can have a notable effect, with metal panels potentially expanding or contracting up to half an inch for every 10 feet due to varying temperatures. This emphasizes the importance of considering thermal expansion during design to maintain structural soundness.
The 2018 IBC also incorporates more stringent specifications for metal panels' resistance to load and deflection, especially in relation to wind loads. This is particularly crucial for areas prone to extreme weather, as it indicates a shift towards ensuring the structural integrity of metal roofs and walls in high-risk environments. The type of metal used, whether it's steel, aluminum, or zinc, influences deflection behavior due to inherent material properties. As such, material selection becomes a crucial factor in meeting design criteria.
When determining deflection limits, engineers are not only concerned with static loads but also with dynamic loads, which include environmental factors like wind and snow. These dynamic forces can alter the structural performance in real-time, requiring a comprehensive understanding of how the structure will react under various conditions. The manufacturing process, including techniques like roll-forming, can have a substantial impact on the warping and deflection of the metal panels. This can create variability in the way different profiles behave under load compared to flat sheets. Continuous panel systems in metal roofing can be beneficial by distributing loads over a broader area, minimizing deflection when compared to older, overlapping systems.
Deflection limits are not simply a structural safety consideration but also influence the aesthetic quality of the roofing system. Excessive deflection can result in unwanted waviness or buckling, which can lead to repair costs or premature replacement. It's crucial to note that the code defines the deflection limit as the amount of flex under the "service load," which includes both the permanent weight of the roof (dead load) and the anticipated variable weights (live load). Design considerations during the planning stage must account for both types of loads to ensure long-term durability.
Further, designers must consider instances of localized deflection that might occur under concentrated loads such as snow accumulation or equipment loads during maintenance. These situations can produce stress points exceeding average design conditions, potentially leading to structural failure if not adequately accounted for. This highlights the need for a thorough engineering assessment throughout the design process to ensure the safety and longevity of metal roof and siding systems.
2018 IBC Analyzing Key Structural Design Updates for Enhanced Building Safety - Live Load Requirements for Secondary Roof Structures
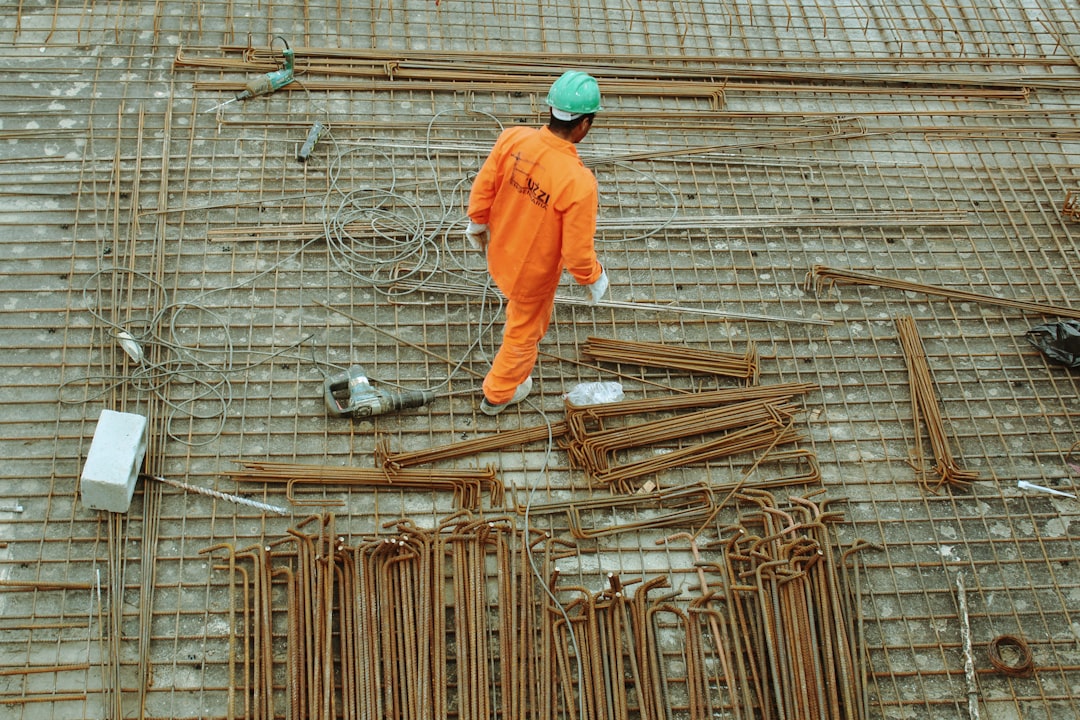
The 2018 International Building Code (IBC) introduces updated requirements for live loads on secondary roof structures, primarily focused on enhancing building safety and performance. These updates place a particular emphasis on the deflection limits of secondary framing members that support formed metal roofs. The code mandates that these members must not deflect beyond specific limits when subjected to live loads. This is not just about meeting code; it’s crucial for maintaining the integrity and aesthetic qualities of the roofing system, especially in areas where dynamic forces like wind and snow are prevalent.
Beyond these specific deflection limitations, the revised code demonstrates a broader focus on building resilience. It acknowledges potential challenges like localized deflection that could occur during extreme weather events. By highlighting the need for rigorous design practices and comprehensive engineering assessments, the IBC aims to ensure that secondary roof structures are adequately prepared to withstand anticipated loads, thereby contributing to the overall safety and longevity of buildings. While some might see these updates as simply adhering to regulations, they are fundamentally about improving the reliability and safety of structures in the face of environmental pressures and material advancements.
The 2018 IBC has brought a more comprehensive approach to live load considerations for secondary roof structures. It acknowledges that loads aren't just static, but encompass dynamic forces like wind and snow, hinting at a deeper understanding of how structures respond to varied conditions. It's fascinating to observe how the building's purpose can influence live load requirements. Roofs intended for areas with high occupancy, such as assembly spaces, require higher live loads compared to areas like storage or service zones. This reflects an emphasis on safety, especially in scenarios with a large number of people.
Furthermore, the code emphasizes the need for accounting for localized effects on secondary roof structures, including things like snow accumulation and maintenance equipment. This necessitates a more detailed analysis beyond general load assumptions during the design process, emphasizing a deeper understanding of potential loading conditions. Beyond the roofing system itself, engineers need to think about the weight of the chosen materials when designing secondary structures. They must factor in loads from mechanical equipment, maintenance, or even green roofs.
Intriguingly, the code suggests that live load design should account for potential future loads. It promotes a forward-thinking approach to design to accommodate shifts in building use or unexpected events. This highlights a focus on long-term structural performance. Interestingly, the code provides flexibility with some reduced load factors for roofs not expected to experience maximum occupancy at all times. This indicates a move toward accommodating real-world conditions while still emphasizing safety.
High-wind regions pose particular challenges for secondary roof structures. While live load requirements address immediate concerns, it's also important that engineers consider how wind forces can interact with these loads, potentially leading to significant changes in load conditions. The increased focus on the relationship between live loads and deflection limits in the 2018 IBC underscores that excessive deflection can lead to not just structural issues, but also increased repair and maintenance costs related to appearance.
The IBC also encourages the use of more advanced modeling methods as part of live load calculations for better prediction under different load scenarios. This is a notable shift towards data-driven engineering practices. It is apparent from the updated live load requirements that continuous education and training for engineers are crucial. Staying abreast of these changes and understanding their implications for structural behavior is critical for maintaining compliance and ensuring the safety of buildings in our constantly evolving construction landscape.
2018 IBC Analyzing Key Structural Design Updates for Enhanced Building Safety - Wall Member Deflection Specifications for Wind Loads
The 2018 International Building Code (IBC) includes new requirements for how wall members handle wind loads, primarily focused on improved safety and building performance. Specifically, secondary wall members that support metal siding are now subject to strict deflection limits under design wind loads, ensuring deflection doesn't exceed a specific span length (l). This change signifies a move towards a more thorough approach to structural design, taking into account both vertical and horizontal deflection. The updated code acknowledges that buildings need to withstand various environmental factors, and these requirements aim to ensure resilience in the face of strong winds and other weather-related issues. The IBC's attention to site-specific conditions like wind speeds and potential localized deflections due to severe weather isn't just about structure; it's also about maintaining the overall look and intended function of the building long-term. This is a noteworthy step in building codes, as designs must now adapt to a more sophisticated understanding of how structures behave when exposed to variable dynamic forces.
The 2018 IBC acknowledges that wall members are subjected to not just constant loads but also changing forces like wind, which adds a layer of complexity to ensuring proper deflection performance. This means designers need to consider a broader range of conditions, including the variability of wind speeds, when evaluating how walls might flex.
Deflection limits within the IBC are directly linked to the total anticipated load a wall might experience. This 'service load' incorporates both the consistent weight of the structure (dead load) and fluctuating loads like snow or temporary equipment used during maintenance. This comprehensive approach to load calculation underscores the importance of considering how all potential forces can impact the behavior of wall members.
One of the intriguing features of the 2018 IBC is its attention to localized deflection. This means that rather than just assuming uniform stress across a wall, designers must acknowledge that certain areas may face greater loads than others, particularly around concentrated forces. This requires a more refined approach to design, ensuring those concentrated load areas are adequately reinforced.
The IBC often defines acceptable deflection as a fraction of the wall's span length, such as L/240. This ratio basically sets the limit for how much sagging or distortion is permissible. It reflects a balance between needing a functional wall and wanting it to look visually appealing.
The type of metal chosen for a wall can have a notable impact on how it deflects under stress. Steel and aluminum behave differently, highlighting the importance of material selection. It's crucial to select a material that meets both safety and performance requirements based on the intended building design.
Another important factor to consider is the effect of temperature changes. Metal expands and contracts significantly – up to half an inch for every 10 feet – leading to shifts in a wall's alignment. It’s essential for engineers to plan for this thermal movement to prevent potential issues related to wall integrity.
The IBC encourages using advanced methods for predicting wall deflection. This move towards more sophisticated modeling is a positive shift, helping engineers produce more precise and resilient structural designs.
In areas with frequent strong winds, the interplay between wind loads and wall deflection becomes especially critical. Excessive flexing under wind stress can weaken materials, ultimately causing expensive repairs or even structural collapse.
The IBC emphasizes that engineers need ongoing training in these updated deflection requirements. This ensures that professionals stay up-to-date with the latest standards, ultimately leading to more reliable and safe building designs.
Finally, excessive wall deflection can be visually obvious, causing a noticeable bowing or distortion. The IBC now places more weight on aesthetics in its requirements, acknowledging that deflection limits are not just a safety issue, but also a factor in the overall appearance and quality of a structure. This indicates a broader view of building design, emphasizing a balance between functionality and appearance.
2018 IBC Analyzing Key Structural Design Updates for Enhanced Building Safety - Aerosol Fire Extinguishing Systems Integration
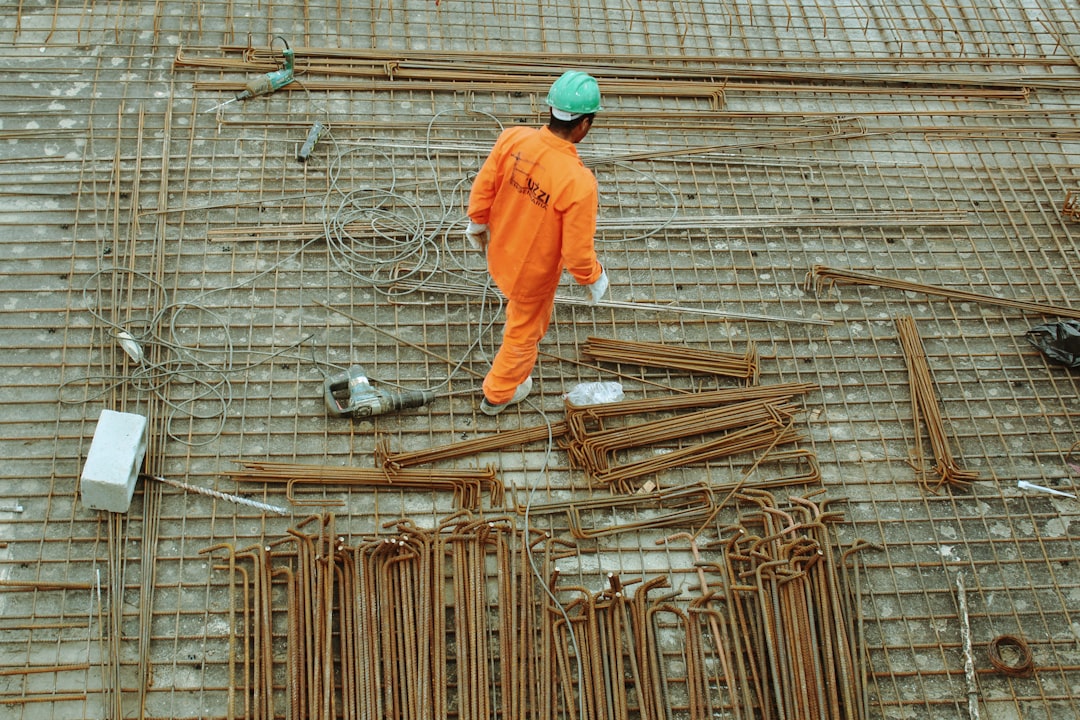
The 2018 International Building Code (IBC) brings aerosol fire extinguishing systems more prominently into building safety regulations. This integration signifies a shift towards incorporating these systems as a core component of fire protection strategies. The IBC outlines that the installation, inspection, maintenance, and testing of these systems should adhere to specific sections within the code, referencing standards and practices aimed at maximizing effectiveness. This includes aligning with other fire protection chapters, which detail a range of aspects like detection, notification, and suppression. The code's emphasis on adhering to manufacturers' guidelines underscores the need for a careful and consistent approach to integration and upkeep. This push for integrating aerosol systems reflects a broader trend in fire safety towards utilizing advanced technologies to improve both response times and the overall efficacy of fire suppression. It's a positive step towards adapting to evolving building types and fire risks. Yet, as with any newly incorporated technology, careful implementation and adherence to all relevant code requirements are critical to ensure the intended safety benefits are realized without introducing unforeseen vulnerabilities.
The 2018 International Building Code (IBC) introduces notable changes related to aerosol fire extinguishing systems, aligning with its overall goal of improving building safety through updated fire protection provisions. Specifically, Section 90414 now directs installers, inspectors, and maintenance personnel to follow both IBC Sections 901 and 904.4, as well as NFPA 2010. This cross-referencing establishes a more comprehensive framework for integrating these systems into building designs.
The IBC, within Chapter 9, has a broad focus on active fire protection. This encompasses a variety of areas, including detection, alarms, and extinguishment. It's interesting how the IBC aims to ensure that buildings are equipped to deal with fires effectively. To verify this, acceptance tests are required, often with a building official present, adhering to the IBC and the International Fire Code. This reinforces the importance of ensuring fire protection systems are up to par, and the 2018 IBC ensures owners bear the cost of these tests.
It’s noteworthy that the IBC emphasizes the significance of adhering to manufacturers' instructions and ensuring systems are listed, a vital aspect of proper integration and ongoing maintenance. The updates in the 2018 IBC can be interpreted as a recognition of technological advancement in fire safety. This includes a wider acceptance of modern technologies, such as aerosol fire suppression, into building designs.
The incorporation of aerosol systems seems to represent a broader movement to modernize fire safety practices. It indicates a potential shift in how the industry approaches building fire protection, adopting the latest technological innovations and standards. It's an interesting area to research further, particularly considering the environmental impact of different fire suppression methods. While there are benefits like potentially minimal residue, one might also wonder if there are unknown risks or drawbacks that future revisions of the code might need to address.
While it might be easy to simply view these changes as a matter of adhering to new rules, a closer look suggests a move towards making building codes more responsive to innovation and the need for better safety in fire protection practices. It's also worth considering how the availability of different systems in different jurisdictions might also impact how these code updates are interpreted and implemented across a variety of locations. Hopefully, as more data on the long-term performance of aerosol systems is collected and evaluated, the code can be further refined to ensure the most effective and safe methods for protecting buildings in the event of a fire.
2018 IBC Analyzing Key Structural Design Updates for Enhanced Building Safety - Mass Timber Construction Provisions
The 2018 International Building Code (IBC) brought about noteworthy changes in how mass timber construction is addressed, emphasizing safety and structural soundness. A key part of this was the creation of three new construction types—IVA, IVB, and IVC—specifically tailored for mass timber buildings. While the existing heavy timber Type IVHT remained untouched, these new types provided a framework for taller timber structures. Later, the 2021 IBC pushed this further, permitting mass timber buildings to reach heights up to 18 stories, subject to specific area limits for each construction type. This evolution highlights the increasing acceptance of mass timber as a viable building material, evidenced by a significant increase in projects in the US and Canada. However, these changes weren't simply about allowing taller buildings. The updated code reflects a cautious approach, with provisions built on thorough fire and life safety testing. As the popularity of mass timber grows, it becomes even more crucial to evaluate the implications for design and safety standards, particularly concerning the inherent risks and fire safety concerns associated with taller timber structures. Finding that careful balance between embracing new methods and ensuring safety remains a vital aspect of the construction industry.
The 2018 International Building Code (IBC) introduced a new set of provisions specifically for mass timber construction, marking a significant shift towards acknowledging the potential of wood as a primary building material. This update allows for the construction of taller timber buildings, reaching heights of up to 18 stories in some cases—a considerable increase compared to earlier codes. This change, arguably, reflects a growing acceptance and understanding of mass timber's strengths, which appear to be overcoming some of the historical concerns around its use in large-scale building projects.
One of the key focuses of the new provisions is on the inherent fire-resistant properties of mass timber. The code acknowledges the charring behavior of materials like cross-laminated timber (CLT) during a fire, highlighting how this characteristic can protect the core structural elements for extended periods.
The IBC also emphasizes the importance of considering how mass timber behaves under lateral loads, such as wind or seismic forces. It seems to mandate a more nuanced structural design approach to ensure stability in both vertical and horizontal directions, highlighting the importance of anticipating these loads for overall safety.
Interestingly, working with mass timber in structural designs requires a deep understanding of the material's properties. This includes how wood's mechanical attributes are affected by species, moisture content, and the natural variation in grain patterns. This seems to highlight the potential for variability that needs careful attention during material selection and structural planning.
Furthermore, the IBC includes specific requirements for how structural connections are made in mass timber buildings. This focus on the connection systems emphasizes their crucial role in load transfer and resisting shear forces, indicating a recognition that those connections are key for maintaining structural integrity.
An appealing aspect of mass timber construction is the potential for prefabrication. This prefabrication process can lead to faster construction times and reduced on-site labor, making it a more efficient approach, though initial design and logistical planning might be more complex.
In addition to embracing new methods, the IBC requires performance-based design reviews for mass timber buildings. This requirement compels engineers to apply rigorous testing and analysis of fire resistance and structural capabilities, prompting them to potentially adopt new methodologies and refine current approaches.
The provisions also encourage the use of advanced modeling techniques to better predict how mass timber structures will respond to loads and environmental factors. This reflects a shift towards a more data-driven, rigorous approach in structural design.
Although mass timber inherently offers aesthetically pleasing elements due to its natural look and texture, the IBC includes updated fire safety guidelines specific to its use. This aspect of the code suggests a careful balancing act between aesthetic freedom in design and ensuring robust fire protection.
The introduction of the mass timber construction provisions appears to be reflective of a wider design trend that sees an increased appreciation for natural materials in construction. This trend seems to be driven by both the performance attributes of these materials and a push for innovative design expression, which is leading to a departure from conventional construction methods.
2018 IBC Analyzing Key Structural Design Updates for Enhanced Building Safety - Structural Observations in Chapter 17
Chapter 17 of the 2018 International Building Code (IBC) emphasizes the need for ongoing structural observations during building construction, a crucial step in ensuring building integrity and safety. These observations, mandated to be performed by qualified professionals like the Engineer of Record or a designated representative, provide an additional layer of quality control. It's important to recognize that these observations don't replace other required inspections, but rather work in conjunction with them, especially in situations where wind or earthquake forces are prominent. While structural observations have been a part of the IBC for a while, this chapter clarifies expectations and reinforces their significance. This emphasis on observation appears to be part of a larger shift in the 2018 IBC that encourages more rigorous testing and analysis, acknowledging that building materials and design techniques have evolved and grown more complex. In essence, these updated requirements within Chapter 17 aim to not simply fulfill code compliance, but to improve overall building performance and safety, ultimately creating a more resilient and dependable built environment.
Chapter 17 of the 2018 International Building Code (IBC) introduces some interesting points regarding structural observations, which are intended to ensure the safety and integrity of buildings during construction. These observations must be carried out by a qualified professional, typically the Engineer of Record or a designated representative. It's worth noting that these observations don't supersede other inspection requirements found in other sections of the code.
These observations, in some form or another, have been a part of building codes for a long time. However, situations involving high winds or earthquakes necessitate them, as outlined in the 2015 IBC. The 2018 version provides updates to wind and seismic hazard maps, likely improving the accuracy of assessing risk in structural design. A new section regarding tsunami loading has been added in Chapter 16, which is a notable inclusion.
We also see revisions in the live load requirements for decks and balconies. Table 1607.1 now mandates a minimum load 15 times higher than usual requirements. The special inspections and tests mentioned in Chapter 17 cover various procedures for verifying materials and construction methods. Any statement detailing these special inspections must include information about the materials, systems, and components, alongside the extent of each required inspection.
One fascinating aspect is the IBC's evolving understanding of the role of real-time monitoring of structural deflection. Sensors that provide real-time data are becoming more common and can help designers compare actual behavior to calculated performance. This opens up opportunities for what some call "adaptive design," where design choices can be fine-tuned based on monitoring the structure's response.
Also intriguing is the increased emphasis on advanced computational tools. Finite Element Analysis (FEA) is increasingly becoming a standard practice. These tools enable a deeper understanding of structural behavior under a wider variety of load scenarios, especially in complex systems.
Additionally, the 2018 IBC places a greater emphasis on the impact of dynamic effects like wind and seismic loads. The new requirements underscore the significance of accurately considering the effects of these factors on structural integrity. The use of computational models in tandem with traditional engineering practices is growing. It seems like a positive direction.
There's also increased focus on considering material variability. This suggests a move towards tailored structural analyses based on specific materials and their characteristics. Localized analysis seems to be getting more attention. Instead of just making broad assumptions, it appears that engineers are being asked to take a closer look at specific zones within a structure that may have higher stresses.
Interestingly, the IBC now calls for the use of historical performance data when developing predictive models. The hope here is to refine the accuracy of how we estimate future risk. Similarly, thermal effects are now explicitly mentioned as a factor that needs to be considered during design. Finally, the 2018 IBC takes a more holistic approach to safety. It suggests that beyond just calculating loads, factors like aesthetics and occupant safety should be considered during the design process, highlighting the idea that structural integrity influences the broader building performance and overall user experience.
It's clear that Chapter 17, within the context of the 2018 IBC, pushes for a more sophisticated, data-driven, and site-specific approach to structural design, taking into account more factors than just the static loads previously considered in the past.
More Posts from financialauditexpert.com: